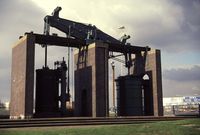

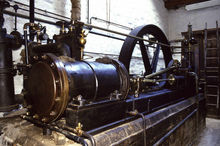

A steam engine is a heat engine that performs mechanical work using steam as its working fluid.
The idea of using boiling water to produce mechanical motion has a long history, going back about 2,000 years. Early devices were not practical power producers, but more advanced designs producing usable power have become a major source of mechanical power over the last 300 years, beginning with applications for removing water from mines using vacuum engines. Subsequent developments using pressurized steam and converting linear to rotational motion enabled the powering of a wide range of manufacturing machinery. This could be sited anywhere that water and coal or wood fuel could be obtained, whereas previous installations were limited to locations where water wheels or windmills could be used. Significantly, this power source would later be applied to prime movers, mobile devices such as steam tractors and railway locomotives. Modern steam turbines generate about 80% of the electric power in the world using a variety of heat sources.[citation needed]
Steam engines are typically external combustion engines,[1] E.C.E.[›] although other external sources of heat such as solar power, nuclear power or geothermal energy may be used. The heat cycle is known as the Rankine cycle.
In general usage, the term 'steam engine' can refer to integrated steam plants such as railway steam locomotives and portable engines, or may refer to the machinery alone, as in the beam engine and stationary steam engine. Specialized devices such as steam hammers and steam pile drivers are dependent on steam supplied from a separate boiler.
History
Following the invention by Denis Papin of the steam digester in 1679, and a first piston steam engine in 1690, the first practical steam-powered 'engine' was a water pump, developed in 1698 by Thomas Savery. It proved only to have a limited lift height and was prone to boiler explosions, but it still received some use for mines and pumping stations.
The first commercially successful engine did not appear until around 1712. Incorporating technologies discovered by Savery and Denis Papin, the atmospheric engine, invented by Thomas Newcomen, paved the way for the Industrial Revolution. Newcomen's engine was relatively inefficient, and in most cases was only used for pumping water. It worked by using the vacuum from condensing steam in a cylinder and was mainly employed for draining mine workings at depths hitherto impossible, but also for providing a reusable water supply for driving waterwheels at factories sited away from a suitable 'head'.
The next major step occurred when James Watt developed (1763–75) an improved version of Newcomen's engine, with a separate condenser. Watt's engine used 75% less coal than Newcomen's, and was hence much cheaper to run. Watt proceeded to develop his engine further, modifying it to provide a rotary motion suitable for driving factory machinery. This enabled factories to be sited away from rivers, and further accelerated the pace of the Industrial Revolution.
Newcomen's and Watt's early engines were "atmospheric", meaning that they were powered by the vacuum generated by condensing steam instead of the pressure of expanding steam. Cylinders had to be large, as the only usable force acting on them was atmospheric pressure. Steam was only used to compensate for the atmosphere allowing the piston to move back to its starting position.
Around 1800, Richard Trevithick introduced engines using high-pressure steam. These were much more powerful than previous engines and could be made small enough for transport applications. Thereafter, technological developments and improvements in manufacturing techniques (partly brought about by the adoption of the steam engine as a power source) resulted in the design of more efficient engines that could be smaller, faster, or more powerful, depending on the intended application.
Steam engines remained the dominant source of power well into the 20th century, when advances in the design of electric motors and internal combustion engines gradually resulted in the vast majority of reciprocating steam engines being replaced in commercial usage, and the ascendancy of steam turbines in power generation.
The history of steam engine development is a vast subject. The following articles cover aspects of steam engine development in greater detail:
- Timeline of steam power – overview
- History of the steam engine – general history, concentrating on reciprocating engines
- Steam turbine – the parallel development of turbine-type engines
- Steam power during the Industrial Revolution
- History of steam road vehicles
The steam cycle
The Rankine cycle is the fundamental thermodynamic underpinning of the steam engine. The Rankine cycle is a cycle that converts heat into work. The heat is supplied externally to a closed loop, which in steam engines contains water and steam. This cycle generates about 80% of all electric power used throughout the world, including virtually all solar thermal, biomass, coal and nuclear power plants. It is named after William John Macquorn Rankine, a Scottish polymath.
The Rankine cycle is sometimes referred to as a practical Carnot cycle because, when an efficient turbine is used, the TS diagram begins to resemble the Carnot cycle. The main difference is that heat addition (in the boiler) and rejection (in the condenser) are isobaric (constant pressure) in the Rankine cycle and isentropic (constant entropy) in the theoretical Carnot cycle. In this cycle a pump is also used to pressurize the working fluid received from the condenser as a liquid instead of as a gas. Pumping the working fluid through the cycle as a liquid requires a very small fraction of the energy needed to transport it as compared to compressing the working fluid as a gas in a compressor (as in the Carnot cycle).
The working fluid in a Rankine cycle follows a closed loop and is reused constantly. While many substances could be used in the Rankine cycle, water is usually the fluid of choice due to its favorable properties, such as nontoxic and unreactive chemistry, abundance, and low cost, as well as its thermodynamic properties.
Components of steam engines
There are two fundamental components of a steam engine: the boiler or steam generator, and the "motor unit", itself often referred to as a "steam engine". The two components can either be integrated into a single unit or can be placed at a distance from each other, in a variety of configurations.
Other components are often present; pumps (such as an injector) to supply water to the boiler during operation, condensers to recirculate the water and recover the latent heat of vaporisation, and superheaters to raise the temperature of the steam above its saturated vapour point, and various mechanisms to increase the draft for fireboxes. When coal is used, a chain or screw stoking mechanism and its drive engine or motor may be included to move the fuel from a supply bin (bunker) to the firebox.
Heat source
The heat required for boiling the water and supplying the steam can be derived from various sources, most commonly from burning combustible materials with an appropriate supply of air in a closed space (called variously combustion chamber, firebox). In some cases the heat source is a nuclear reactor or geothermal energy.
Boilers
Boilers are pressure vessels that contain water to be boiled, and some kind of mechanism for transferring the heat to the water so as to boil it.
The two most common methods of transferring heat to the water according are:
- water-tube boiler - water is contained in or run through one or several tubes surrounded by hot gases
- fire-tube boiler - the water partially fills a vessel below or inside which is a combustion chamber or furnace and fire tubes through which the hot gases flow
Once turned to steam, many boilers raise the temperature of the steam further, turning 'wet steam' into 'superheated steam'. This use of superheating prevents the steam condensing within the engine, and allows significantly greater efficiency.
Motor units
A motor unit takes a supply of steam at high pressure and temperature and gives out a supply of steam at lower pressure and temperature, using as much of the difference in steam energy as possible to do mechanical work.
A motor unit is often called 'steam engine' in its own right. They will also operate on compressed air or other gas.
Simple expansion
This means that a charge of steam works only once in the cylinder. It is then exhausted directly into the atmosphere or into a condenser, but remaining heat can be utilized if needed to heat a living space, or to provide warm feedwater for the boiler.
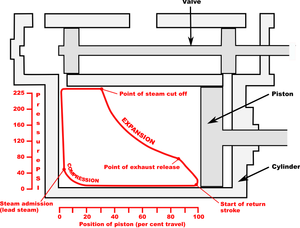

In most reciprocating piston engines, the steam reverses its direction of flow at each stroke (counterflow), entering and exhausting from the cylinder by the same port. The complete engine cycle occupies one rotation of the crank and two piston strokes; the cycle also comprises four events — admission, expansion, exhaust, compression. These events are controlled by valves often working inside a steam chest adjacent to the cylinder; the valves distribute the steam by opening and closing steam ports communicating with the cylinder end(s) and are driven by valve gear, of which there are many types. The simplest valve gears give events of fixed length during the engine cycle and often make the engine rotate in only one direction. Most however have a reversing mechanism which additionally can provide means for saving steam as speed and momentum are gained by gradually "shortening the cutoff" or rather, shortening the admission event; this in turn proportionately lengthens the expansion period. However, as one and the same valve usually controls both steam flows, a short cutoff at admission adversely affects the exhaust and compression periods which should ideally always be kept fairly constant; if the exhaust event is too brief, the totality of the exhaust steam cannot evacuate the cylinder, choking it and giving excessive compression ("kick back").
In the 1840s and 50s, there were attempts to overcome this problem by means of various patent valve gears with a separate, variable cutoff expansion valve riding on the back of the main slide valve; the latter usually had fixed or limited cutoff. The combined setup gave a fair approximation of the ideal events, at the expense of increased friction and wear, and the mechanism tended to be complicated. The usual compromise solution has been to provide lap by lengthening rubbing surfaces of the valve in such a way as to overlap the port on the admission side, with the effect that the exhaust side remains open for a longer period after cut-off on the admission side has occurred. This expedient has since been generally considered satisfactory for most purposes and makes possible the use of the simpler Stephenson, Joy and Walschaerts motions. Corliss, and later, poppet valve gears had separate admission and exhaust valves driven by trip mechanisms or cams profiled so as to give ideal events; most of these gears never succeeded outside of the stationary marketplace due to various other issues including leakage and more delicate mechanisms.[6][7]
- Compression
Before the exhaust phase is quite complete, the exhaust side of the valve closes, shutting a portion of the exhaust steam inside the cylinder. This determines the compression phase where a cushion of steam is formed against which the piston does work whilst its velocity is rapidly decreasing; it moreover obviates the pressure and temperature shock, which would otherwise be caused by the sudden admission of the high pressure steam at the beginning of the following cycle.
- Lead
The above effects are further enhanced by providing lead: as was later discovered with the internal combustion engine, it has been found advantageous since the late 1830s to advance the admission phase, giving the valve lead so that admission occurs a little before the end of the exhaust stroke in order to fill the clearance volume comprising the ports and the cylinder ends (not part of the piston-swept volume) before the steam begins to exert effort on the piston.[8]
Oscillating cylinder steam engines
Compounding engines
A method to lessen the magnitude of this heating and cooling was invented in 1804 by British engineer Arthur Woolf, who patented his Woolf high pressure compound engine in 1805. In the compound engine, high pressure steam from the boiler expands in a high pressure (HP) cylinder and then enters one or more subsequent lower pressure (LP) cylinders. The complete expansion of the steam now occurs across multiple cylinders and as less expansion now occurs in each cylinder so less heat is lost by the steam in each. This reduces the magnitude of cylinder heating and cooling, increasing the efficiency of the engine. To derive equal work from lower pressure steam requires a larger cylinder volume as this steam occupies a greater volume. Therefore the bore, and often the stroke, are increased in low pressure cylinders resulting in larger cylinders.
Double expansion (usually known as compound) engines expanded the steam in two stages. The pairs may be duplicated or the work of the large LP cylinder can be split with one HP cylinder exhausting into one or the other, giving a 3-cylinder layout where cylinder and piston diameter are about the same making the reciprocating masses easier to balance.
Two-cylinder compounds can be arranged as:
- Cross compounds - The cylinders are side by side.
- Tandem compounds - The cylinders are end to end, driving a common connecting rod
- Angle compounds - The cylinders are arranged in a vee (usually at a 90° angle) and drive a common crank.
With two-cylinder compounds used in railway work, the pistons are connected to the cranks as with a two-cylinder simple at 90° out of phase with each other (quartered). When the double expansion group is duplicated, producing a 4-cylinder compound, the individual pistons within the group are usually balanced at 180°, the groups being set at 90° to each other. In one case (the first type of Vauclain compound), the pistons worked in the same phase driving a common crosshead and crank, again set at 90° as for a two-cylinder engine. With the 3-cylinder compound arrangement, the LP cranks were either set at 90° with the HP one at 135° to the other two, or in some cases all three cranks were set at 120°.
The adoption of compounding was common for industrial units, for road engines and almost universal for marine engines after 1880; it was not universally popular in railway locomotives where it was often perceived as complicated. This is partly due to the harsh railway operating environment and limited space afforded by the loading gauge (particularly in Britain, where compounding was never common and not employed after 1930). However although never in the majority it was popular in many other countries.[6]
Multiple expansion engines
It is a logical extension of the compound engine (described above) to split the expansion into yet more stages to increase efficiency. The result is the multiple expansion engine. Such engines use either three or four expansion stages and are known as triple and quadruple expansion engines respectively. These engines use a series of double-acting cylinders of progressively increasing diameter and/or stroke and hence volume. These cylinders are designed to divide the work into three or four, as appropriate, equal portions for each expansion stage. As with the double expansion engine, where space is at a premium, two smaller cylinders of a large sum volume may be used for the low pressure stage. Multiple expansion engines typically had the cylinders arranged inline, but various other formations were used. In the late 19th century, the Yarrow-Schlick-Tweedy balancing 'system' was used on some marine triple expansion engines. Y-S-T engines divided the low pressure expansion stages between two cylinders, one at each end of the engine. This allowed the crankshaft to be better balanced, resulting in a smoother, faster-responding engine which ran with less vibration. This made the 4-cylinder triple-expansion engine popular with large passenger liners (such as the Olympic class), but was ultimately replaced by the virtually vibration-free turbine (see below).
The image to the right shows an animation of a triple expansion engine. The steam travels through the engine from left to right. The valve chest for each of the cylinders is to the left of the corresponding cylinder.
Land-based steam engines could exhaust much of their steam, as feed water was usually readily available. Prior to and during World War I, the expansion engine dominated marine applications where high vessel speed was not essential. It was however superseded by the British invention steam turbine where speed was required, for instance in warships, such as the dreadnought battleships, and ocean liners. HMS Dreadnought of 1905 was the first major warship to replace the proven technology of the reciprocating engine with the then-novel steam turbine.
Uniflow (or unaflow) engine
This is intended to remedy the difficulties arising from the usual counterflow cycle mentioned above which means that at each stroke the port and the cylinder walls will be cooled by the passing exhaust steam, whilst the hotter incoming admission steam will waste some of its energy in restoring working temperature. The aim of the uniflow is to remedy this defect by providing an additional port uncovered by the piston at the end of each stroke making the steam flow only in one direction. By this means, thermal efficiency is improved by having a steady temperature gradient along the cylinder bore. The simple-expansion uniflow engine is reported to give efficiency equivalent to that of classic compound systems with the added advantage of superior part-load performance. It is also readily adaptable to high-speed uses and was a common way to drive electricity generators towards the end of the 19th century before the coming of the steam turbine.
All common steam admission valves, such as slide valves, piston valves and rotary Corliss type valves, have been used on uniflow engines, usually actuated by common eccentrics. The most advanced uniflow engines used poppet valves, which allow high steam inlet temperatures. The inlet valves may be driven by a double cam system whose phasing and duration is controllable; this allows adjustments for high torque and power when needed with more restrained use of steam and greater expansion for economical cruising.
Uniflow engines have been produced in single-acting, double-acting, simple, and compound versions. Skinner 4-crank 8-cylinder single-acting tandem compound [9] engines power two Great Lakes ships still trading today (2007). These are the Saint Marys Challenger,[10] that in 2005 completed 100 years of continuous operation as a powered carrier (the Skinner engine was fitted in 1950) and the car ferry, SS Badger.[11]
In the early 1950s, the Ultimax engine — a 2-crank 4-cylinder arrangement similar to Skinner's — was developed by Abner Doble for the Paxton car project with tandem opposed single-acting cylinders giving effective double-action.[12]
Small uniflow steam engines have been made as conversions of two-stroke internal combustion engines, by feeding the cylinder with steam via a "bash valve" in the spark plug hole which is knocked open by the piston reaching the top of its stroke.[13]
Turbine engines
Steam turbines provide direct rotational force and therefore do not require a linkage mechanism to convert reciprocating to rotary motion. Thus, they produce smoother rotational forces on the output shaft. This contributes to a lower maintenance requirement and less wear on the machinery they power than a comparable reciprocating engine.
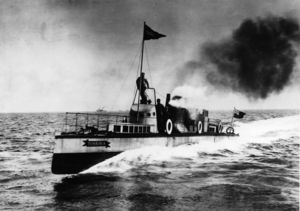

The main use for steam turbines is in electricity generation (about 80% of the world's electric production is by use of steam turbines)[citation needed] and to a lesser extent as marine prime movers. In the former, the high speed of rotation is an advantage, and in both cases the relative bulk is not a disadvantage; in the latter (pioneered on the Turbinia), the light weight, high efficiency and high power are highly desirable.
Virtually all nuclear power plants generate electricity by heating water to provide steam that drives a turbine connected to an electrical generator. Nuclear-powered ships and submarines either use a steam turbine directly for main propulsion, with generators providing auxiliary power, or else employ turbo-electric propulsion, where the steam drives a turbine-generator set with propulsion provided by electric motors. A limited number of steam turbine railroad locomotives were manufactured. Some non-condensing direct-drive locomotives did meet with some success for long haul freight operations in Sweden and for express passenger work in Britain, but were not repeated. Elsewhere, notably in the U.S.A., more advanced designs with electric transmission were built experimentally, but not reproduced. It was found that steam turbines were not ideally suited to the railroad environment and these locomotives failed to oust the classic reciprocating steam unit in the way that modern diesel and electric traction has done.
Rotary steam engines
It is possible to use a mechanism based on a pistonless rotary engine such as the Wankel engine in place of the cylinders and valve gear of a conventional reciprocating steam engine. Many such engines have been designed, from the time of James Watt to the present day, but relatively few were actually built and even fewer went into quantity production; see link at bottom of article for more details. The major problem is the difficulty of sealing the rotors to make them steam-tight in the face of wear and thermal expansion; the resulting leakage made them very inefficient. Lack of expansive working, or any means of control of the cutoff is also a serious problem with many such designs. By the 1840s, it was clear that the concept had inherent problems and rotary engines were treated with some derision in the technical press. However, the arrival of electricity on the scene, and the obvious advantages of driving a dynamo directly from a high-speed engine, led to something of a revival in interest in the 1880s and 1890s, and a few designs had some limited success.
Of the few designs that were manufactured in quantity, those of the Hult Brothers Rotary Steam Engine Company of Stockholm, Sweden, and the spherical engine of Beauchamp Tower are notable. Tower's engines were used by the Great Eastern Railway to drive lighting dynamos on their locomotives, and by the Admiralty for driving dynamos on board the ships of the Royal Navy. They were eventually replaced in these niche applications by steam turbines.
Jet type
Invented by Australian engineer Alan Burns and developed in Britain by engineers at Pursuit Dynamics, this underwater jet engine uses high pressure steam to draw in water through an intake at the front and expel it at high speed through the rear. The engine can also serve as pump and mixer. This type of system is referred to as 'PDX Technology' by Pursuit Dynamics and has been applied to food technology problems.[14]
Rocket type
In more modern times there has been limited use of steam for rocketry—particularly for rocket cars. The technique is simple in concept, simply fill a pressure vessel with hot water at high pressure, and open a valve leading to a suitable nozzle. The drop in pressure immediately boils some of the water and the steam leaves through a nozzle, giving a significant propulsive force.
There are even speculative plans for interplanetary use. Although steam rockets are relatively inefficient in their use of propellant, this very well may not matter as the solar system is believed to have extremely large stores of water ice which can be used as propellant. Extracting this water and using it in interplanetary rockets requires several orders of magnitude less equipment than breaking it down to hydrogen and oxygen for conventional rocketry.[15]
Cold sink
As with all heat engines, a considerable quantity of waste heat at relatively low temperature is produced and must be disposed of.
The simplest cold sink is to vent the steam to the environment. This is often used on steam locomotives, as the released steam is released in the chimney so as to increase the draw on the fire, which greatly increases engine power, but is inefficient. Steam locomotive condensing apparatus can be employed to improve efficiency.
Sometimes the waste heat is useful in and of itself, and in those cases very high overall efficiency can be obtained. For example, combined heat and power (CHP) systems use the waste steam for district heating.
Where CHP is not used, steam turbines in power stations use surface condensers as a cold sink. The condensers are cooled by water flow from oceans, rivers, lakes, and often by cooling towers which evaporate water to provide cooling energy removal. The resulting condensed hot water output from the condenser is then put back into the boiler via a pump. The water vapor with entrained droplets often seen billowing from power stations is generated by the cooling systems (not from the closed-loop Rankine power cycle) and represents the waste energy heat (pumping and vaporization) that could not be converted to useful work in the turbine. Note that cooling towers operate using the latent heat of vaporization of the cooling fluid. The white billowing clouds that form in cooling tower operation are the result of water droplets that are entrained in the cooling tower airflow; they are not, as commonly thought, released steam.
Water pump
The Rankine cycle and most practical steam engines have a water pump to recycle or top up the boiler water, so that they may be run continuously. The water pump can be of almost any type although special types, such as an injector pump, which is a pump that uses a steam jet usually supplied from the boiler and is present on very many steam locomotives.
Monitoring equipment
For safety reasons, nearly all steam engines are equipped with mechanisms to monitor the boiler, such as a pressure gauge and a sight glass to monitor the water level.
Many engines, stationary and mobile, are also fitted with a governor to regulate the speed of the engine without the need for human interference (similar to cruise control in some cars).
Advantages
The strength of the steam engine for modern purposes is in its ability to convert heat from almost any source into mechanical work, unlike the internal combustion engine.
Similar advantages are found in a different type of external combustion engine, the Stirling engine, which can offer efficient power (with advanced regenerators and large radiators) at the cost of a much lower power-to-size/weight ratio than even modern steam engines with compact boilers[citation needed]. These Stirling engines are not commercially produced, although the concepts are promising.
Steam locomotives are especially advantageous at high elevations as they are not adversely affected by the lower atmospheric pressure. This was inadvertently discovered when steam locomotives operated at high altitudes in the mountains of South America were replaced by diesel-electric units of equivalent sea level power. These were quickly replaced by much more powerful locomotives capable of producing sufficient power at high altitude.
For road vehicles, steam propulsion has the advantage of having high torque from stationary, removing the need for a clutch and transmission, though start-up time and sufficiently compact packaging remain a problem.
In Switzerland (Brienz Rothhorn) and Austria (Schafberg Bahn) new rack steam locomotives have proved very successful. They were designed based on a 1930s design of Swiss Locomotive and Machine Works (SLM) but with all of today's possible improvements like roller bearings, heat insulation, light-oil firing, improved inner streamlining, one-man-driving and so on. These resulted in 60 percent lower fuel consumption per passenger[citation needed] and massively reduced costs for maintenance and handling[citation needed]. Economics now are similar or better than with most advanced diesel or electric systems. Also a steam train with similar speed and capacity is 50 percent lighter than an electric or diesel train[citation needed], thus, especially on rack railways, significantly reducing wear and tear on the track. Also, a new steam engine for a paddle steam ship on Lake Geneva, the Montreux, was designed and built, being the world's first full-size ship steam engine with an electronic remote control.[16] The steam group of SLM in 2000 created a wholly owned company called DLM to design modern steam engines and steam locomotives.
Safety
Steam engines possess boilers and other components that are pressure vessels that contain a great deal of potential energy. Steam escapes and boiler explosions (typically BLEVEs) can and have caused great loss of life in the past. While variations in standards may exist in different countries, stringent legal, testing, training, care with manufacture, operation and certification is applied to try to minimise or prevent such occurrences.
Failure modes may include:
- over-pressurisation of the boiler
- insufficient water in the boiler causing overheating and vessel failure
- pressure vessel failure of the boiler due to inadequate construction or maintenance.
- escape of steam from pipework/boiler causing scalding
Steam engines frequently possess two independent mechanisms for ensuring that the pressure in the boiler does not go too high; one may be adjusted by the user, the second is typically designed as an ultimate fail-safe.
Lead fusible plugs may be present in the crown of the firebox. If the water level drops, such that the temperature of the firebox crown increases significantly, the lead melts and the steam escapes warning the operators, who may then manually drop the fire. Except in the smallest of boilers the steam escape has little effect on dampening the fire. The plugs are also too small in area to lower steam pressure significantly, depressurizing the boiler. If they were any larger, the volume of escaping steam would itself endanger the crew.
Efficiency
No heat engine can be more efficient than the Carnot cycle, in which heat is moved from a high temperature reservoir to one at a low temperature, and the efficiency depends on the temperature difference. For the greatest efficiency, steam engines should be operated at the highest steam temperature possible (superheated steam), and release the waste heat at the lowest temperature possible.
The efficiency of a Rankine cycle is usually limited by the working fluid. Without the pressure reaching super critical levels for the working fluid, the temperature range the cycle can operate over is quite small; in steam turbines, turbine entry temperatures are typically 565°C (the creep limit of stainless steel) and condenser temperatures are around 30°C. This gives a theoretical Carnot efficiency of about 63% compared with an actual efficiency of 42% for a modern coal-fired power station. This low turbine entry temperature (compared with a gas turbine) is why the Rankine cycle is often used as a bottoming cycle in combined-cycle gas turbine power stations.
One of the principal advantages the Rankine cycle holds over others is that during the compression stage relatively little work is required to drive the pump, the working fluid being in its liquid phase at this point. By condensing the fluid, the work required by the pump consumes only 1% to 3% of the turbine power and contributes to a much higher efficiency for a real cycle. The benefit of this is lost somewhat due to the lower heat addition temperature. Gas turbines, for instance, have turbine entry temperatures approaching 1500°C. Nonetheless, the efficiencies of actual large steam cycles and large modern gas turbines are fairly well matched.
In practice, a steam engine exhausting the steam to atmosphere will typically have an efficiency (including the boiler) in the range of 1-10%, but with the addition of a condenser and multiple expansion, it may be greatly improved to 25% or better.
A megawatt electrical power station with steam reheat, economizer etc. will achieve up to 50% thermal efficiency.[17]
It is also possible to capture the waste heat using cogeneration in which the waste heat is used for heating a lower boiling point working fluid or as a heat source for district heating via saturated low pressure steam. By this means it is possible to use as much as 85-90% of the input energy.
Applications
Since the early 18th century, steam power has been applied to a variety of practical uses. At first it was applied to reciprocating pumps, but from the 1780s rotative engines (i.e. those converting reciprocating motion into rotary motion) began to appear, driving factory machinery such as spinning mules and power looms. At the turn of the 19th century, steam-powered transport on both sea and land began to make its appearance becoming ever more dominant as the century progressed.
Steam engines can be said to have been the moving force behind the Industrial Revolution and saw widespread commercial use driving machinery in factories, mills and mines; powering pumping stations; and propelling transport appliances such as railway locomotives, ships and road vehicles. Their use in agriculture led to an increase in the land available for cultivation.
Very low power engines are used to power models and speciality applications such as the steam clock.
The presence of several phases between heat source and power delivery has meant that it has always been difficult to obtain a power-to-weight ratio anywhere near that obtainable from internal combustion engines; notably this has made steam aircraft extremely rare. Similar considerations have meant that for small and medium-scale applications steam has been largely superseded by internal combustion engines or electric motors, which has given the steam engine an out-dated image. However it is important to remember that the power supplied to the electric grid is predominantly generated using steam turbine plant, so that indirectly the world's industry is still dependent on steam power. Recent concerns about fuel sources and pollution have incited a renewed interest in steam both as a component of cogeneration processes and as a prime mover. This is becoming known as the Advanced Steam movement.
Steam engines can be classified by their application:
Stationary applications
Stationary steam engines can be classified into two main types:
- Winding engines, rolling mill engines, steam donkeys, marine engines, and similar applications which need to frequently stop and reverse.
- Engines providing power, which rarely stop and do not need to reverse. These include engines used in thermal power stations and those that were used in pumping stations, mills, factories and to power cable railways and cable tramways before the widespread use of electric power.
The steam donkey is technically a stationary engine but is mounted on skids to be semi-portable. It is designed for logging use and can drag itself to a new location. Having secured the winch cable to a sturdy tree at the desired destination, the machine will move towards the anchor point as the cable is winched in.
A portable engine is a stationary engine mounted on wheels so that it may be towed to a work-site by horses or a traction engine, rather than being fixed in a single location.
Transport applications
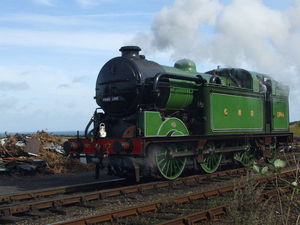

Steam engines have been used to power a wide array of transport appliances:
- Marine: Steamboat, steamship, steam yacht
- Rail: Steam locomotive, fireless locomotive
- Agriculture: Traction engine, steam tractor
- Road: Steam wagon, steam bus, steam tricycle, steam car
- Construction: Steam roller, steam shovel
- Military: steam tank (tracked), steam tank (wheeled), steam catapult
- Space: Steam rocket
In these applications internal combustion engines are now used due to their higher power-to-weight ratio , lower maintenance and space requirements .
Modern applications
The company Energiprojekt AB in Sweden has made progress in using modern materials for harnessing the power of steam. The efficiency of Energiprojekt's steam engine reaches some 27-30% on high-pressure engines. It is a single-step, 5-cylinder engine (no compound) with superheated steam and consumes approx. 4 kg (8.8 lb) of steam per kWh.[18]
This article is copied from an article on Wikipedia® - the free encyclopedia created and edited by online user community. The text was not checked or edited by anyone on our staff. Although the vast majority of the Wikipedia® encyclopedia articles provide accurate and timely information please do not assume the accuracy of any particular article. This article is distributed under the terms of GNU Free Documentation License.
Thanks to Farlex, Inc. / Encyclopedia The Free Dictionary
http://encyclopedia.thefreedictionary.com/p/Steam%20engine
No comments:
Post a Comment